
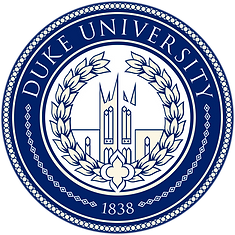
GREG WANG LABORATORY
@ Duke University
Department of Pharmacology and Cancer Biology
Department of Pathology (adjunct)
Duke Cancer Institute
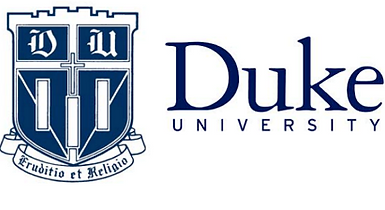


Research
With an emphasis on chromatin biology and gene regulation, our research programs are broadly focused on mechanistic understandings of how chemical modifications of chromatin define distinctive patterns of mammalian genomes, control gene expression and regulate cell fates during development, and how their deregulations lead to human disease such as cancer, developmental disorder and aging.
Routinely, we have taken a set of integrated biochemical and biophysical, genomics, oncology and medicinal chemistry approaches to tackle the broad and critical questions in this field. Often, inter-laboratory and interdisciplinary collaborations are instrumental in successful carryout of our projects.
Our interest can roughly be summarized into the following research directions:
(I) Histone modification ‘reader’ proteins in gene regulation and pathogenesis.
Utilizing biochemical approaches as a tool of discovery, my laboratory and colleagues have contributed to identification of different domain classes that specifically bind various histone modifications. We believe that these histone modification ‘readers’ are crucially involved in gene/genome regulation, cell fate decision-making and development.
Fig 1. Structure of KDM5A’s PHD finger (A) and PHF1’s Tudor domain (B) in complex with H3K4me3 and H3K36me3, respectively.
In the past, we have studied a set of ‘reader’ proteins specific for H3K4me3 (Fig 1A), a prominent gene-activation histone mark. Several of these H3K4me3 ‘reader’-encoding genes show recurrent alterations (e.g. mutations or chromosomal rearrangement) in disease, notably acute leukemia. Works of us and collaborators are among the first to demonstrate that deregulation of histone methylation ‘readers’ is causal for initiating cancer (Nature 2009; Mol Cell 2010; Cancer Discovery 2014; Nat comm 2020). Furthermore, we have discovered a histone methylation-‘reading’ activity harbored within Polycomb-like proteins, PHF1 (Fig 1B) and PHF19, which modulates genome-targeting of PRC2, a crucial gene-repressive protein complex, in stem cells (Mol. Cell 2013). Polycomb-like protein family genes are frequently mutated and altered due to either chromosomal rearrangement or over-expression in human cancer (Blood 2019).
Fig 2. BAH domain (left) and chromo domain (right) based functional readout of H3K27me3 in gene silencing.
Trimethylated histone H3 lysine 27 (H3K27me3) regulates gene repression, cell-fate determination and differentiation. More recently, we report that a conserved bromo-adjacent homology (BAH) module of BAHCC1 (BAHCC1 BAH; Fig 2 left) 'recognizes' H3K27me3 specifically and enforces silencing of H3K27me3-demarcated genes in mammalian cells (Nature Genetics 2020). BAHCC1 is highly expressed in human acute leukemia and interacts with transcriptional corepressors. In leukemia, depletion of BAHCC1, or disruption of the BAHCC1 BAH-H3K27me3 interaction, causes derepression of H3K27me3-targeted genes that are involved in tumor suppression and cell differentiation, leading to suppression of oncogenesis. In mice, introduction of a germline mutation at BAHCC1 BAH to disrupt its H3K27me3 engagement causes partial postnatal lethality, supporting a role in development. This study identifies an H3K27me3-directed transduction pathway in mammals that relies on a conserved BAH 'reader' (Nature Genetics 2020). Similar BAH motifs also exist among other 'readers' in the mammals (such as BAHD1; Nucleic Acid Res 2021), plants and fungi, indicating an evolutionary conservation of the BAH based functional readout of H3K27me3 during gene silencing.
In future, we will continue to characterize additional histone ‘readers’ we identified in the contexts of both fundamental chromatin biology and their relevance to disease, especially cancer. A far-reaching implication of our research is that histone ‘reader’ proteins are potentially druggable. Bromodomain inhibitors such as JQ1 make a strong argument. Partnership with structural biologists and medicinal chemists allows us to develop into this area further.
Conceptually, we favor a view that human diseases notably cancer often arise from mis-regulation of a ‘histone language’ embedded in cells’ epi-genomes when it is either mis-‘written’, mis-‘interpreted’ or mis-‘erased’, a view that we have been active in putting forward with a set of timely, comprehensive review articles (Mut Res. 2008; Trends in Mol Med 2007; Nature Rev Cancer 2010; Trends in Bio Chem. 2013; Blood 2015; Exp. Hematology 2015; Frontier in Oncol. 2017; Cellular and Molecular Life Sciences, 2019; Trend Genet 2021; Nat Rev Cancer 2021).
(II) Phase separation underlying the gene regulation and 3D chromatin organization.
Transcription factors (TFs) are essential for gene regulation and three dimensional (3D) genome organization. How the events of TF binding, DNA looping (such as enhancer:promoter loops) and gene transcription are coordinated still remain far from clear. Recent studies point to an involvement of phase separation, a phenomenon that refers to membrane-less organelles or cellular condensates formed often via biomolecular liquid–liquid phase separation (LLPS).
We recently investigated into human haematological malignancies characterized by recurrent chromosomal translocation of nucleoporin (NUP98 or NUP214), which generates an aberrant chimera that invariably retains the nucleoporin IDR— tandemly dispersed repeats of phenylalanine and glycine residues (FG repeats). How do unstructured IDRs contribute to oncogenesis? We report that IDRs contained within NUP98–HOXA9, a homeodomain containing transcription factor chimera recurrently detected in leukaemias, are essential for establishing liquid–liquid phase separation (LLPS) puncta of chimera and for inducing leukaemic transformation. Consequently, LLPS of NUP98–HOXA9 not only promotes chromatin occupancy of chimera transcription factors, but also is required for the formation of a broad ‘super-enhancer’-like binding pattern typically seen at leukaemogenic genes, which potentiates transcriptional activation. Deeply sequenced Hi-C revealed that phase-separated NUP98–HOXA9 induces CTCF-independent chromatin loops that are enriched at proto-oncogenes. Together, this report describes a proof-of-principle example in which cancer acquires mutation to establish oncogenic transcription factor condensates via phase separation, which simultaneously enhances their genomic targeting and induces organization of aberrant three-dimensional chromatin structure during tumourous transformation. This work was done in collaboration with Drs. Doug Phanstiel and Wes Legant at UNC and others.
As LLPS-competent molecules are frequently implicated in diseases, this mechanism can potentially be generalized to many malignant and pathological settings.
(III) DNA methylation machineries in epigenetic regulation and pathogenesis.
DNA methylation represents another epigenetic mechanism important for gene/genome regulation, organismal development, and tumorigenesis. For example, DNMT3A, the gene encoding a de novo methyltransferase, was recently identified as one of the most frequently mutated genes among patients with various hematological malignancies (especially acute myeloid leukemia) and old individuals with clonal hematopoiesis.
Fig 3. A model showing cooperativity between the mutations of DNMT3A and proliferative kinases during AML progression.
In this area, we have recently used murine models to show that somatic DNMT3A mutation often acts in concert with kinase mutation (such as RAS) to promote AML development (Fig 3). Mechanistically, these AML-related DNMT3A mutations do not cause global DNA methylation alterations but rather focal hypomethylation of CpG sites enriched in cis-regulatory elements (notably enhancers). These CpG hypomethylations interfere with differentiation-associated silencing of enhancers that control key genes known to be critical for AML or hematopoietic stem cell expansion. Importantly, such an epigenetic alteration relies on DOT1L complexes for gene activation (Fig 3), which renders AML cells a superior sensitivity to DOT1L inhibitors (Cancer Cell 2016; Oncoscience 2016). Currently, we are using different cancer models that range from cancer cell lines, GEM models and human PDX models to further test this pathway, in a hope to develop the mechanism-based therapeutics. Additionally, we have worked with a long-term structural biology collaborator (Dr. Jikui Song) and solved the first DNMT3A-CpG complex structure (Nature 2018). This work not only reveals how DNMT3A mediates de novo DNA methylation but also shows how cancer-related DNMT3A mutations affect substrate binding to promote malignant transformation (Fig 4). Along the same lines of DNA methylation, our labs also study DNMT1 (Nat comm 2021; PNAS 2020) and DNMT3b (Nat comm 2020).
Fig 4. (Top) Patterns of CpG versus non-CpG methylation change due to a substitution of a DNA-binding amino acid of DNMT3A. (Bottom) Cancer cells acquire mutation (in blue) at the DNMT3A’s DNA binding sites.
(IV) Chemical inhibition of epigenetic regulators, leading to new therapeutics.
Discovery of small-molecule inhibitors to target histone-modifying enzymes or ‘readers’ has become an area of intensive investigation and holds great promise for new therapies. In this research direction, we have worked closely with medicinal chemists at UNC (Drs. Jian Jin and Stephen Frye) who developed the first orally bioavailable, highly potent and selective inhibitor (termed as UNC1999) for EZH2 and related EZH1 enzymes (Fig 5, left); further, we have shown UNC1999’s cancer-suppressive effects in vitro and in animal models of acute leukemias (ACS Chem biol 2013; Blood 2015). Currently, we are continuing to develop the so-called “degrader” epigenetic inhibitors via PROTAC, which both inhibit and degrade target proteins in tumor cells.
Fig 5. Left: Docking of UNC1999 on EZH2. Right: Design and development of EZH2/1 degrader using UNC1999 and PROTAC.
In addition, we investigated into aberrant androgen receptor (AR) signaling, which contributes to therapy resistance seen in patients with castration-resistant prostate cancer, the most common tumor in men; here we not only identified a new cofactor ZFX-related control of AR signaling but, importantly, showed BRD4 inhibitors to be effective in suppressing aberrant AR/ZFX signaling in prostate tumor (Fig 6; Mol Cell 2018).
Fig 6. ZFX Mediates Non-canonical Oncogenic Functions of the Androgen Receptor Splice Variant 7 in Castrate-Resistant Prostate Cancer
Towards this direction of research, our goal is to yield drug candidates with preclinical cancer models we have established in the research laboratories, which shall pave the way for translating the novel therapeutic approach in the clinic (with assistance of clinical trial specialists for these future directions).
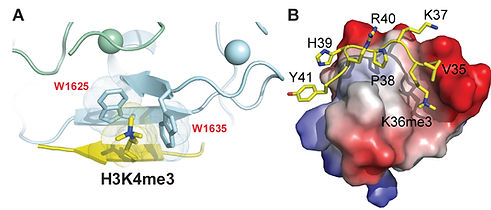
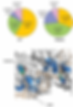
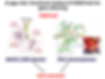



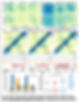
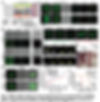

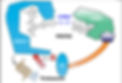